基于蜡烛灰构建的电-Fenton体系中H2O2的生成与磺胺甲恶唑的降解
H2O2 in-situ production and sulfamethoxazole degradation in the electro-Fenton system constructed with candle soots
-
摘要: 本文构建了基于蜡烛灰(CS)的电-Fenton体系,研究了体系中H2O2的原位生成和磺胺甲恶唑(SMX)的降解.研究发现,在蜡烛火焰中部收集得到的CS为40-50 nm的疏水性球状颗粒.经过热处理后,样品的比表面积由61.792 m2·g-1(CS)升高到67.098 m2·g-1(CS-200)、102.54 m2·g-1(CS-400)、322.85 m2·g-1(CS-600)和680.69 m2·g-1(CS-800),为氧气还原反应(ORR)提供了更多的反应活性位点.介孔体积由0.1463 cm3·g-1(CS)升高到0.1723 cm3·g-1(CS-200)、0.1740 cm3·g-1(CS-400)、0.2686 cm3·g-1(CS-600)、和0.3570 cm3·g-1(CS-800).除CS-200外,热处理增加了样品表面含氧官能团比例,显著提高了样品的亲水性.旋转圆盘电极(RDE)实验证明,CS,CS-200和CS-400表面ORR为2e-途径,而CS-600和CS-800表面氧气还原涉及4e-途径,并且与其他样品相比,CS-400显示出最高的2e- ORR催化活性,这与其表面存在较高比例的COOH基团(23.15%)和较大的介孔比例(50.39%)有关.在以CS-400空气阴极搭建的电-Fenton体系中,H2O2浓度在60 min内可以积累到1277 mg·L-1,产量可达5.11 mg·h-1·cm-2.连续5次SMX降解实验中,60 min内SMX的降解率均保持在100%.因此,基于CS构建的电-Fenton体系显示出很高的SMX降解能力和运行稳定性,具有很大的实际应用价值.Abstract: In this paper, an electro-Fenton system based on candle soot (CS) was constructed. The in situ production of H2O2 and the degradation of sulfamethoxazole (SMX) were studied. Based on the experiments, the pristine CS collected in the middle of the candle flame was hydrophobic spheres. After heating treatment, the specific surface area of the samples increased from 61.792 m2·g-1 (CS) to 67.098 m2·g-1 (CS-200), 102.54 m2·g-1 (CS-400), 322.85 m2·g-1 (CS-600), and 680.69 m2·g-1 (CS-800), and the mesopore volumes increased from 0.1463 cm3·g-1 (CS) to 0.1723 cm3·g-1 (CS-200), 0.1740 cm3·g-1 (CS-400), 0.2686 cm3·g-1 (CS-600), and 0.3570 cm3·g-1 (CS-800). The increased specific surface area provided more active sites for the reaction of oxygen reduction (ORR). Except CS-200, the content of oxygen-containing functional groups increased after the heating treatment, which significantly improved the hydrophilicity of the samples. Rotating disk electrode (RDE) experiments showed that oxygen was reduced through 2e-pathway on the surfaces of CS, CS-200, and CS-400, while 4e- pathway was involved in the oxygen reduction on the surfaces of the CS-600 and CS-800. Compared with other samples, CS-400 exhibited the highest of 2e- ORR activity, which can be explained by the highest content of COOH groups (23.15%) and mesopores ratio (50.39%). In the electro-Fenton system, H2O2 reached its maximum concentration of 1277 mg·L-1 with a yield of 5.11 mg·h-1·cm-2 in 60 min. In five consecutive degradation experiments, the degradation rates of SMX all remained at 100% within 60 min. Therefore, the electro-Fenton system based on CS exhibited high SMX degradation ability and operating stability, which endowed it with a great potential for practical application.
-
Key words:
- electro-Fenton /
- H2O2 /
- oxygen reduction reaction (ORR) /
- two electron path /
- sulfamethoxazole
-
-
[1] 陈姗, 许凡, 张玮, 等.磺胺类抗生素污染现状及其环境行为的研究进展[J].环境化学, 2019, 38(7):1557-1569. CHEN S, XU F, ZHANG W, et al. Research progress in pollution situation and environmental behavior of Sulfonamides[J].Environmental Chemistry,2019,38(7):1557-1569(in Chinese).
[2] LUO Y, XU L, RYSZ M, et al. Occurrence and transport of tetracycline, sulfonamide, quinolone, and macrolide antibiotics in the Haihe River basin, China[J]. Environmental Science & Technology, 2011, 45(5):1827-1833. [3] JIANG L, HU X, YIN D, et al. Occurrence, distribution and seasonal variation of antibiotics in the Huangpu River, Shanghai, China[J]. Chemosphere, 2011, 82(6):822-828. [4] 洪蕾洁, 石璐, 张亚雷, 等. 固相萃取-高效液相色谱法同时测定水体中的10种磺胺类抗生素[J].环境科学,2012,33(2):652-657. HONG L J, SHI L, ZHANG Y L, et al.Simultaneous determination of 10 sulfonamide antibiotics in water by solid phase extraction and high performance liquid chromatograph[J]. Environmental Science,2012,33(2):652-657(in Chinese).
[5] VALIM R B, REIS R M, CASTRO P S, et al. Electrogeneration of hydrogen peroxide in gas diffusion electrodes modified with tert-butyl-anthraquinone on carbon black support[J]. Carbon, 2013, 61:236-244. [6] VISWANATHAN V, HANSEN H A, ROSSMEISL J, et al. Unifying the 2e(-) and 4e(-) reduction of oxygen on metal surfaces[J]. Journal of Physical Chemistry Letters, 2012, 3(20):2948-2951. [7] JIRKOVSKY J S, PANAS I, AHLBERG E, et al. Single atom Hot-spots at Au-Pd nanoalloys for electrocatalytic H2O2 production[J]. Journal of the American Chemical Society, 2011, 133(48):19432-19441. [8] PIZZUTILO E, KASIAN O, CHOI C H, et al. Electrocatalytic synthesis of hydrogen peroxide on Au-Pd nanoparticles:From fundamentals to continuous production[J]. Chemical Physics Letters, 2017, 683:436-442. [9] FREAKLEY S J, HE Q, HARRHY J H, et al. Palladium-tin catalysts for the direct synthesis of H2O2 with high selectivity[J]. Science, 2016, 351(6276):965-968. [10] IGLESIAS D, GIULIANI A, MELCHIONNA M, et al. N-doped graphitized carbon nanohorns as a forefront electrocatalyst in highly selective O2 reduction to H2O2[J]. Chem, 2018, 4(1):106-123. [11] GUO D, SHIBUYA R, AKIBA C, et al. Active sites of nitrogen-doped carbon materials for oxygen reduction reaction clarified using model catalysts[J]. Science, 2016, 351(6271):361-365. [12] KHATAEE A R, SAFARPOUR M, ZAREI M, et al. Electrochemical generation of H2O2 using immobilized carbon nanotubes on graphite electrode fed with air:Investigation of operational parameters[J]. Journal of Electroanalytical Chemistry, 2011, 659(1):63-68. [13] HAN L, SUN Y, LI S, et al. In-plane carbon lattice-defect regulating electrochemical oxygen reduction to hydrogen peroxide production over nitrogen-doped graphene[J]. ACS Catalysis, 2019, 9(2):1283-1288. [14] PARK J, NABAE Y, HAYAKAWA T, et al. Highly selective two-electron oxygen reduction catalyzed by mesoporous nitrogen-doped carbon[J]. Acs Catalysis, 2014, 4(10):3749-3754. [15] SUN Y, SINEV I, JU W, et al. Efficient electrochemical hydrogen peroxide production from molecular oxygen on nitrogen-doped mesoporous carbon catalysts[J]. ACS Catalysis, 2018, 8(4):2844-2856. [16] YANG Y, HE F, SHEN Y, et al. A biomass derived N/C-catalyst for the electrochemical production of hydrogen peroxide[J]. Chemical Communications, 2017, 53(72):9994-9997. [17] ZHAO H, SHEN X, CHEN Y, et al. A COOH-terminated nitrogen-doped carbon aerogel as a bulk electrode for completely selective two-electron oxygen reduction to H2O2[J]. Chemical Communications, 2019, 55(44):6173-6176. [18] KAKUNURI M, SHARMA C S. Candle soot derived fractal-like carbon nanoparticles network as high-rate lithium ion battery anode material[J]. Electrochimica Acta, 2015, 180:353-359. [19] KHALAKHAN I, FIALA R, LAVKOVA J, et al. Candle soot as efficient support for proton exchange membrane fuel cell catalyst[J]. Fuel Cells, 2016, 16(5):652-655. [20] SINGH S, BAIRAGI P K, VERMA N. Candle soot-derived carbon nanoparticles:An inexpensive and efficient electrode for microbial fuel cells[J]. Electrochimica Acta, 2018, 264:119-127. [21] LIANG C J, LIAO J D, LI A J, et al. Relationship between wettabilities and chemical compositions of candle soots[J]. Fuel, 2014, 128:422-427. [22] ZHANG Z, HAO J, YANG W, et al. Modifying candle soot with FeP nanoparticles into high-performance and cost-effective catalysts for the electrocatalytic hydrogen evolution reaction[J]. Nanoscale, 2015, 7(10):4400-4405. [23] ZHOU M, LI Q, ZHONG S, et al. Facile large scale fabrication of magnetic carbon nano-onions for efficient removal of bisphenol A[J]. Materials Chemistry and Physics, 2017, 198:186-192. [24] XIAO L, ZENG W, LIAO G, et al. Thermally and chemically stable candle soot superhydrophobic-surface with excellent self-cleaning properties in air and qil[J]. Acs Applied Nano Materials, 2018, 1(3):1204-1211. [25] CAO H, FU J, LIU Y, et al. Facile design of superhydrophobic and superoleophilic copper mesh assisted by candle soot for oil water separation[J]. Colloids and Surfaces a-Physicochemical and Engineering Aspects, 2018, 537:294-302. [26] YUAN S, STROBBE D, KRUTH J-P, et al. Super-hydrophobic 3D printed polysulfone membranes with a switchable wettability by self-assembled candle soot for efficient gravity-driven oil/water separation[J]. Journal of Materials Chemistry A, 2017, 5(48):25401-25409. [27] PANKAJ A, TEWARI K, SINGH S, et al. Waste candle soot derived nitrogen doped carbon dots based fluorescent sensor probe:An efficient and inexpensive route to determine Hg(II) and Fe (III) from water[J]. Journal of Environmental Chemical Engineering, 2018, 6(4):5561-5569. [28] SUN W, ZHANG X, JIA H R, et al. Water-dispersible candle soot-derived carbon nano-onion clusters for imaging-guided photothermal cancer therapy[J]. Small, 2019, 15(11):1804575. [29] ROCHE I, SCOTT K. Carbon-supported manganese oxide nanoparticles as electrocatalysts for oxygen reduction reaction (ORR) in neutral solution[J]. Journal of Applied Electrochemistry, 2009, 39(2):197-204. [30] DONG H, YU H, WANG X, et al. A novel structure of scalable air-cathode without Nafion and Pt by rolling activated carbon and PTFE as catalyst layer in microbial fuel cells[J]. Water Research, 2012, 46(17):5777-5787. [31] LIANG L, ZHOU M, LU X, et al. High-efficiency electrogeneration of hydrogen peroxide from oxygen reduction by carbon xerogels derived from glucose[J]. Electrochimica Acta, 2019, 320:134569. [32] CHEN S, CHEN Z, SIAHROSTAMI S, et al. Defective carbon-based materials for the electrochemical synthesis of hydrogen peroxide[J]. ACS Sustainable Chemistry & Engineering, 2018, 6(1):311-317. [33] ZHU J, XIAO X, ZHENG K, et al. KOH-treated reduced graphene oxide:100% selectivity for H2O2 electroproduction[J]. Carbon, 2019, 153:6-11. [34] MIAO J, ZHU H, TANG Y, et al. Graphite felt electrochemically modified in H2SO4 solution used as a cathode to produce H2O2 for pre-oxidation of drinking water[J]. Chemical Engineering Journal, 2014, 250:312-318. [35] KIM M, OH I, KIM J. Effects of different electrolytes on the electrochemical and dynamic behavior of electric double layer capacitors based on a porous silicon carbide electrode[J]. Physical Chemistry Chemical Physics, 2015, 17(25):16367-16374. [36] YU F, TAO L, CAO T. High yield of hydrogen peroxide on modified graphite felt electrode with nitrogen-doped porous carbon carbonized by zeolitic imidazolate framework-8(ZIF-8) nanocrystals[J]. Environmental Pollution, 2019, 255(Pt 2):113119. [37] WANG C T, HU J L, CHOU W L, et al. Removal of color from real dyeing wastewater by electro-Fenton technology using a three-dimensional graphite cathode[J]. Journal of Hazardous Materials, 2008, 152(2):601-606. [38] REIS R M, BEATI A A G F, ROCHA R S, et al. Use of gas diffusion electrode for the in situ generation of hydrogen peroxide in an electrochemical flow-by reactor[J]. Industrial & Engineering Chemistry Research, 2012, 51(2):649-654. [39] AN J, LI N, ZHAO Q, et al. Highly efficient electro-generation of H2O2 by adjusting liquid-gas solid three phase interfaces of porous carbonaceous cathode during oxygen reduction reaction[J]. Water Research, 2019, 164,114933. [40] LU X, ZHOU M, LI Y, et al. Improving the yield of hydrogen peroxide on gas diffusion electrode modified with tert-butyl-anthraquinone on different carbon support[J]. Electrochimica Acta, 2019, 320,134552. [41] LIU H, WANG C, LI X, et al. A novel electro-Fenton process for water treatment:Reaction-controlled pH adjustment and performance assessment[J]. Environmental Science & Technology, 2007, 41(8):2937-2942. [42] QIANG Z M, CHANG J H, HUANG C P. Electrochemical regeneration of Fe2+ in Fenton oxidation processes[J]. Water Research, 2003, 37(6):1308-1319. -
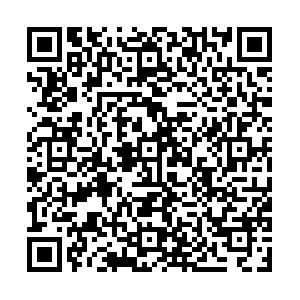
计量
- 文章访问数: 1337
- HTML全文浏览数: 1337
- PDF下载数: 62
- 施引文献: 0