抗生素的胁迫与抗生素抗性基因产生与传播关系的研究
The Relationship of Antibiotic Stress with Emergence and Dissemination of Antibiotic Resistance Genes
-
摘要: 抗生素的环境残留和抗生素抗性基因(antibiotic resistance genes,ARGs)污染日益增加,对全球公共卫生构成重大威胁。目前,关于环境中抗生素与ARGs产生与传播的关系的研究较多,但结果却不尽相同。为了确定抗生素的胁迫与ARGs产生与传播的关系,用磺胺类抗生素(SAs)对大肠杆菌(Escherichia coli,E.coli)毒性作用表征SAs的胁迫作用,用突变和接合转移表征ARGs的产生与传播,测定了SAs对大肠杆菌的毒性、突变频率和结合转移频率的影响,根据剂量-效应曲线,计算了毒性参数(无观察效应浓度(NOEC)、抑制率为50%的化合物浓度(EC50)、抑制率为80%的化合物浓度(EC80)),突变效应参数(促进率为1%时最低可观测突变促进效应浓度(MC0-1)、促进率为50%时突变促进效应浓度(MC50)、促进率最大时突变促进效应浓度(MCmax))和接合转移效应参数(促进率为1%时最低可观测接合转移促进效应浓度(RC0-1)、促进率最大时接合转移促进效应浓度(RCmax)和促进率为1%时最高可观测接合转移促进效应浓度(RC0-2)),利用线性回归分析的方法探究SAs的胁迫与大肠杆菌突变频率和结合转移频率之间的关系,并分析其可能的机制。结果表明,磺胺的高胁迫作用导致核苷酸碱基的大量减少,在DNA复制与转录时,碱基对错配的概率大大增加,从而开始促进突变频率。SAs的低胁迫作用可能引起大肠杆菌的SOS反应,SOS反应可以上调质粒编码的基因以及控制细胞膜的通透性基因,从而提高其接合转移频率。此外,真实环境中存在许多其他的因素也会影响ARGs的产生和传播,据此,本文建议在探索真实环境中ARGs的产生和传播时,应考虑真实环境中其他影响因素和抗生素胁迫的综合作用。上述研究为探索抗生素胁迫对ARGs产生与传播的影响提供了新的思路。Abstract: The pollution induced by antibiotic residues and antibiotic resistance genes (ARGs) has been significantly threating the global public health. Although the relationship between antibiotics and ARGs in the environment have been studied by many investigators, there is no unified conclusion for these results. To investigate the relationship of antibiotics stress with the emergence and dissemination of ARGs, the effects of sulfonamides (SAs) on growth, mutation frequency and conjugative transfer frequency were determined using Escherichia coli (E. coli) as a target organism in this study. The SAs stress on E. coli was expressed as the toxic effects of SAs on the growth of E. coli, and the emergence and dissemination of ARGs were characterized by the mutation and conjugative transfer, respectively. The typical mutation parameters, including minimum observable concentration of SAs (MC0-1), the concentration of SAs that induces the 50% promotion (MC50) and maximum promotion (MCmax) were respectively selected to describe the onset of stimulation, the moderate stimulation, and the maximum stimulation effect of mutation frequency. The typical conjugative transfer parameters, including the minimum observable concentration of SAs (RC0-1), the concentration of SAs that induces the maximum promotion (RCmax), and the maximum observable concentration of SAs (RC0-2) were respectively selected to describe the onset of stimulation, the maximum stimulation, and the terminal stimulation effect of conjugative transfer frequency. The linear regression was conducted to analyze the relationship of the stress of SAs with the mutation frequency and conjugative transfer frequency in E. coli and explore their possible mechanism. The results show that the high stress of SAs results in a substantial reduction in the number of nucleotide bases, which increases the probability of base pair mismatch during DNA replication and transcription, thus promoting the mutation frequency. The low stress of SAs may trigger the SOS response in E. coli, which promotes the conjugative transfer frequency by up-regulating the genes encoded by plasmid and controlling the membrane permeability. Previous studies suggested that many complicated influence factors in the real environment might impact the emergence and dissemination of ARGs, such as the type of compound, concentration of the compound, and transmitting medium. Therefore, it is necessary to comprehensively consider these possible factors when exploring the relationship of antibiotic stress with the emergence and dissemination of ARGs. The present research proposes a new insight into the emergence and dissemination of ARGs under the stress of antibiotics.
-
Key words:
- antibiotics /
- sulfonamides /
- antibiotic resistance genes /
- mutation /
- conjugative transfer
-
-
Managaki S, Murata A, Takada H, et al. Distribution of macrolides, sulfonamides, and trimethoprim in tropical waters:Ubiquitous occurrence of veterinary antibiotics in the Mekong Delta[J]. Environmental Science & Technology, 2007, 41(23):8004-8010 Jiang L, Hu X, Yin D, et al. Occurrence, distribution and seasonal variation of antibiotics in the Huangpu River, Shanghai, China[J]. Chemosphere, 2011, 82(6):822-828 Qin Y, Wen Q, Ma Y, et al. Antibiotics pollution in Gonghu Bay in the period of water diversion from Yangtze River to Taihu Lake[J]. Environmental Earth Sciences, 2018, 77(11):419 Li Y, Wu X, Mo C, et al. Investigation of sulfonamide, tetracycline, and quinolone antibiotics in vegetable farmland soil in the Pearl River Delta Area, Southern China[J]. Journal of Agricultural and Food Chemistry, 2011, 59(13):7268-7276 Hartmann E M, Hickey R, Hsu T, et al. Antimicrobial chemicals are associated with elevated antibiotic resistance genes in the indoor dust microbiome[J]. Environmental Science & Technology, 2016, 50(18):9807-9815 Zhang Q, Ying G, Pan C, et al. Comprehensive evaluation of antibiotics emission and fate in the river basins of China:Source analysis, multimedia modeling, and linkage to bacterial resistance[J]. Environmental Science & Technology, 2015, 49(11):6772-6782 Wright G D. Antibiotic resistance in the environment:A link to the clinic?[J]. Current Opinion In Microbiology, 2010, 13(5):589-594 Tripathi V, Tripathi P. Antibiotic Resistance Genes:An Emerging Environmental Pollutant[M]. Kesari K K. Environmental Science and Engineering. Springer, 2017:183-201 Li S, Shi W, Liu W, et al. A duodecennial national synthesis of antibiotics in China's major rivers and seas (2005-2016)[J]. Science of the Total Environment, 2018, 615:906-917 Ghosh S, Lapara T M. The effects of subtherapeutic antibiotic use in farm animals on the proliferation and persistence of antibiotic resistance among soil bacteria[J]. The ISME Journal, 2007, 1(3):191-203 Zhao R, Feng J, Liu J, et al. Deciphering of microbial community and antibiotic resistance genes in activated sludge reactors under high selective pressure of different antibiotics[J]. Water Research, 2019, 151:388-402 Zhang Q, Jia A, Wan Y, et al. Occurrences of three classes of antibiotics in a natural river basin:Association with antibiotic-resistant Escherichia coli[J]. Environmental Science & Technology, 2014, 48(24):14317-14325 Rodriguez-Mozaz S, Chamorro S, Marti E, et al. Occurrence of antibiotics and antibiotic resistance genes in hospital and urban wastewaters and their impact on the receiving river[J]. Water Research, 2015, 69:234-242 Hurst J J, Oliver J P, Schueler J, et al. Trends in antimicrobial resistance genes in manure blend pits and long-term storage across dairy farms with comparisons to antimicrobial usage and residual concentrations[J]. Environmental Science & Technology, 2019, 53(5):2405-2415 Jia J, Guan Y, Cheng M, et al. Occurrence and distribution of antibiotics and antibiotic resistance genes in Ba River, China[J]. Science of the Total Environment, 2018, 642:1136-1144 Martinez J L, Baquero F. Mutation frequencies and antibiotic resistance[J]. Antimicrobial Agents and Chemotherapy, 2000, 44(7):1771-1777 Davison J. Genetic exchange between bacteria in the environment[J]. Plasmid, 1999, 42(2):73-91 谢志雄, 沈萍. 细菌遗传转化与水平基因转移[J]. 中南民族大学学报:自然科学版, 2003(4):1-5 Xie Z X, Shen P. Bacterial genetic transformation and horizontal gene transfer[J]. Journal of Central South University for Nationalities:Natural Science, 2003 (4):1-5(in Chinese)
Solomon J M, Grossman A D. Who's competent and when:Regulation of natural genetic competence in bacteria[J]. Trends in Genetics, 1996, 12(4):150-155 黄锦岭. 水平基因转移及其发生机制[J]. 科学通报, 2017, 62(12):1221-1225 Huang J L. Horizontal gene transfer and its mechanism[J]. Chinese Science Bulletin, 2017, 62(12):1221-1225(in Chinese)
Lerminiaux N A, Cameron A D S. Horizontal transfer of antibiotic resistance genes in clinical environments[J]. Canadian Journal of Microbiology, 2019, 65(1):34-44 Zhou L, Ying G, Liu S, et al. Excretion masses and environmental occurrence of antibiotics in typical swine and dairy cattle farms in China[J]. Science of the Total Environment, 2013, 444:183-195 Hall B M, Ma C, Liang P, et al. Fluctuation analysis calculator:A web tool for the determination of mutation rate using Luria-Delbruck fluctuation analysis[J]. Bioinformatics, 2009, 25(12):1564-1565 Seydel J K. Sulfonamides, structure-activity relationship, and mode of action. Structural problems of the antibacterial action of 4-aminobenzoic acid (PABA) antagonists[J]. Journal of Pharmaceutical Sciences, 1968, 57(9):1455-1478 Brown G M. The biosynthesis of folic acid. Ⅱ. Inhibition by sulfonamides[J]. The Journal of Biological Chemistry, 1962, 237(6):536-540 Skold O. Sulfonamide resistance:Mechanisms and trends[J]. Drug Resistance Updates, 2000, 3(3):155-160 Wang T, Liu Y, Wang D, et al. The joint effects of sulfonamides and quorum sensing inhibitors on Vibrio fischeri:Differences between the acute and chronic mixed toxicity mechanisms[J]. Journal of Hazardous Materials, 2016, 310:56-67 Vedantam G, Guay G G, Austria N E, et al. Characterization of mutations contributing to sulfathiazole resistance in Escherichia coli[J]. Antimicrobial Agents and Chemotherapy, 1998, 42(1):88-93 Sultan I, Rahman S, Jan A T, et al. Antibiotics, resistome and resistance mechanisms:A bacterial perspective[J]. Frontiers in Microbiology, 2018, 9:2066 Andersson D I, Hughes D. Microbiological effects of sublethal levels of antibiotics[J]. Nature Reviews Microbiology, 2014, 12(7):465-478 Thomas C M, Nielsen K M. Mechanisms of, and barriers to, horizontal gene transfer between bacteria[J]. Nature Reviews Microbiology, 2005, 3(9):711-721 Sorensen S J, Bailey M, Hansen L H, et al. Studying plasmid horizontal transfer in situ:A critical review[J]. Nature Reviews Microbiology, 2005, 3(9):700-710 Wang Y, Lu J, Mao L, et al. Antiepileptic drug carbamazepine promotes horizontal transfer of plasmid-borne multi-antibiotic resistance genes within and across bacterial genera[J]. The ISME Journal, 2019, 13(2):509-522 Zhang Y, Gu A Z, Cen T, et al. Sub-inhibitory concentrations of heavy metals facilitate the horizontal transfer of plasmid-mediated antibiotic resistance genes in water environment[J]. Environmental Pollution, 2018, 237:74-82 Bae Y S, Oh H, Rhee S G, et al. Regulation of reactive oxygen species generation in cell signaling[J]. Molecules and Cells, 2011, 32(6):491-509 Schieber M, Chandel N S. ROS function in redox signaling and oxidative stress[J]. Current Biology, 2014, 24(10):R453-R462 Xie Y, Wei Y, Shen Y, et al. TADB 2.0:An updated database of bacterial type Ⅱ toxin-antitoxin loci[J]. Nucleic Acids Research, 2018, 46(D1):D749-D753 Harms A, Maisonneuve E, Gerdes K. Mechanisms of bacterial persistence during stress and antibiotic exposure[J]. Science, 2016, 354(6318):f4268 Maslowska K H, Makiela Dzbenska K, Fijalkowska I J. The SOS system:A complex and tightly regulated response to DNA damage[J]. Environmental and Molecular Mutagenesis, 2019, 60(4):368-384 Kim S, Yun Z, Ha U, et al. Transfer of antibiotic resistance plasmids in pure and activated sludge cultures in the presence of environmentally representative micro-contaminant concentrations[J]. Science of the Total Environment, 2014, 468-469:813-820 Hu J, Shi J, Chang H, et al. Phenotyping and genotyping of antihiotic-resistant Escherichia coli isolated from a natural river basin[J]. Environmental Science & Technology, 2008, 42(9):3415-3420 -
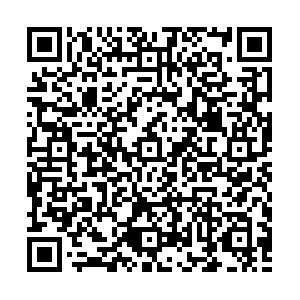
计量
- 文章访问数: 6229
- HTML全文浏览数: 6229
- PDF下载数: 189
- 施引文献: 0