腐殖质电子传递机制及其环境效应研究进展
Electron transfer mechanisms of humic substances and their environmental implications:A review
-
摘要: 腐殖质通过充当微生物的电子受体和氧化物的电子供体可以加速微生物与胞外电子受体间的电子传递速率.腐殖质的电子传递能力受自身结构、来源和外界条件等多种因素的影响.腐殖质不仅能够很大程度地加速电子传递进程,且具有结构和性质稳定等特点,是自然环境中较理想的电子穿梭体.与经过化学提取、纯化后的腐殖质相比较,土壤固相腐殖质更能反映实际环境中的电子传递过程.目前,已有很多研究将腐殖质的电子传递应用于土壤污染治理、温室效应的缓解和水污染处理等多个领域.但在腐殖质电子传递机制及其环境应用的研究中还存在诸多不足,需要广大学者做更进一步的探究.Abstract: Humic substances(HS) can serve as electron shuttles that promote the electron transfer from microorganisms to extracellular electron acceptors. The electron transfer capacity of HS could be influenced by many factors, including molecular structure, source, and environmental conditions. HS can not only accelerate the process of electron transfer, but also have stable structure and chemical properties, and this is why HS can be used as an ideal electron shuttle in the natural environments. Compared with dissolved HS which are extracted and purified by chemical reagents, the solid phase HS could better reflect the electron transfer processes in the real environment. In this review article, we highlight recent advances in the understanding of the applications of HS on the remediation of soil pollution, the mitigation of greenhouse effect, and the treatment of water pollution. Although many researches have been conducted on electron transfer via HS, some issues with respect to the electron transfer mechanism of HS and its environment implications should be addressed further.
-
-
[1] STEVENSON F J. Humus chemistry:genesis, composition, reactions[M]. 2nd Edition. New York:Wiley, 1994:17. [2] LOVLEY D R, COATES J D, BLUNT-HARRIS E L, et al. Humic substances as electron acceptors for microbial respiration[J]. Nature, 1996, 382(6590):445-448. [3] PIEPENBROCK A, SCHRÖDER C, KAPPLER A. Electron transfer from humic substances to biogenic and abiogenic Fe(Ⅲ) oxyhydroxide minerals[J]. Environmental Science & Technology, 2014, 48(3):1656-1664. [4] MARTINEZ C M, ALVAREZ L H, CELIS L B, et al. Humus-reducing microorganisms and their valuable contribution in environmental processes[J]. Applied Microbiology & Biotechnology, 2013, 97(24):10293-10308. [5] NEVIN K P, LOVLEY D R. Potential for nonenzymatic reduction of Fe(Ⅲ) via electron shuttling in subsurface sediments[J]. Environmental Science & Technology, 2000, 34(12):2472-2478. [6] JIANG J, KAPPLER A. Kinetics of microbial and chemical reduction of humic substances:implications for electron shuttling[J]. Environmental Science & Technology, 2008, 42(10):3563-3569. [7] RODEN E, KAPPLER A, BAUER I, et al. Extracellular electron transfer through microbial reduction of solid-phase humic substances[J]. Nature Geoscience, 2010, 3(6):417-421. [8] ZHANG C F, KATAYAMA A. Humin as an electron mediator for microbial reductive dehalogenation[J]. Environmental Science & Technology, 2012, 46(12):6575-6583. [9] VAN DER ZEE F P, CERVANTES F J. Impact and application of electron shuttles on the redox(bio)transformation of contaminants:A review[J]. Biotechnology Advances, 2009, 27(3):256-277. [10] KAPPLER A, STRAUB K L. Geomicrobiological cycling of iron[J]. Reviews in Mineralogy & Geochemistry, 2005, 59(1):85-108. [11] WEBER K A, ACHENBACH L A, COATES J D. Microorganisms pumping iron:Anaerobic microbial iron oxidation and reduction[J]. Nature Reviews Microbiology, 2006, 4(10):752-764. [12] REGUERA G, MCCARTHY K D, MEHTA T, et al. Extracellular electron transfer via microbial nanowires[J]. Nature, 2005, 435(7045):1098-1101. [13] 许杰龙,周顺桂,袁勇,等. 有"生命"的电线:浅析微生物纳米导线电子传递机制及其应用[J]. 化学进展,2012,24(9):1794-1800. XU J L, ZHOU X G, YUAN Y, et al. Live wire:A review on electron transfer mechanism and applications of microbial nanowires[J]. Progress in Chemistry, 2012, 24(9):1794-1800(in Chinese).
[14] HARRIS H W, EL-NAGGAR M Y, BRETSCHGER O, et al. Electrokinesis is a microbial behavior that requires extracellular electron transport[J]. Proceedings of the National Academy of Sciences, 2010, 107(1):326-331. [15] LOVLEY D R, WOODWARD J C, CHAPELLE F H. Stimulated anoxic biodegradation of aromatic hydrocarbons using Fe(Ⅲ) ligands[J]. Nature, 1994, 370(6485):128-131. [16] ALVAREZ L H and CERVANTES F J.(Bio)nanotechnologies to enhance environmental quality and energy production[J]. Journal of Chemical Technology and Biotechnology, 2011, 86:1354-1363. [17] KAPPLER A, BENZ M, SCHINK B, et al. Electron shuttling via humic acids in microbial iron(Ⅲ) reduction in a freshwater sediment[J]. FEMS Microbiology Ecology, 2004. 47(1):85-92. [18] PERETYAZHKO T, SPOSITO G. Reducing capacity of terrestrial humic acids[J]. Geoderma, 2006, 137(1-2):140-146. [19] VISSER S A. Oxidation-reduction potentials and capillary activities of humic acids[J]. Nature, 1964, 204(495):581. [20] BENZ M, SCHINK B, BRUNE A. Humic acid reduction by Propionibacterium freudenreichii and other fermenting bacteria[J]. Applied and Environment Microbiology, 1998, 64(11):4507-4512. [21] LOVLEY D R, FRAGA J L, BLUNT-HARRIS E L, et al. Humic substances as a mediator for microbially catalyzed metal reduction[J]. Acta Hydrochimica et Hydrobiologica, 1998, 26(3):152-157. [22] LOVLEY D R, FRAGA J L, COATES J D, et al. Humics as an electron donor for anaerobic respiration[J]. Environmental Microbiology, 1999, 1(1):89-98. [23] KAPPLER A, HADERLEIN S B. Natural organic matter as reductant for chlorinated aliphatic pollutants[J]. Environmental Science & Technology, 2003, 37(12):2714-2719. [24] AESCHBACHER M, SANDER M, SCHWARZENBACH R P. Novel electrochemical approach to assess the redox properties of humic substances[J]. Environmental Science & Technology, 2010, 44(1):87-93. [25] GESCHER J S, CORDOVA C D, SPORMANN A M. Dissimilatory iron reduction in Escherichia coli:identification of cymA of Shewanella oneidensis and napC of E-coli as ferric reductases[J]. Molecular Microbiology, 2008, 68(3):706-719. [26] LIES D P, HERNANDEZ M E, KAPPLER A, et al. Shewanella oneidensis MR-1 uses overlapping pathways for iron reduction at a distance and by direct contact under conditions relevant for biofilms[J]. Applied and Environment Microbiology, 2005, 71(8):4414-4426. [27] VOORDECKERS J W, KIM B C, IZALLALEN M, et al. Role of Geobacter sulfurreducens outer surface c-type cytochromes in reduction of soil humic acid and anthraquinone-2,6-disulfonate[J]. Applied and Environment Microbiology, 2010, 76(7):2371-2375. [28] PIEPENBROCK A, KAPPLER A. Humic substance and extracellular electron transfer. In Microbial Metal Respiration:From geochemistry to potential applications[M]. Germany:Springer, 2012:107-128. [29] KULIKOVA N A, PERMINOVA I V, BADUN G A, et al. Estimation of uptake of humic substances from different sources by E.coli cells under optimum and salt stress conditions estimated with a use of tritium-labeled humic materials[J]. Applied and Environment Microbiology, 2010, 76(18):6223-6230. [30] SHYU J B H, LIES D P, NEWMAN D K. Protective role of tolC in efflux of the electron shuttle anthraquinone-2,6-disulfonate[J]. Journal of Bacteriology, 2002, 184(6):1806-1810. [31] 马晨,周顺桂,庄莉,等. 微生物胞外呼吸电子传递机制研究进展[J]. 生态学报,2011,31(7):2008-2018. MA C, ZHOU S G, ZHUANG L, et al. Electron transfer mechanism of extracellular respiration:A review[J]. Acta Ecologica Sinica, 2011, 31(7):2008-2018(in Chinese).
[32] CLARKE T A, EDWARDS M J, GATES A J, et al. Structure of a bacterial cell surface decaheme electron conduit[J]. Proceedings of the National Academy of Sciences, 2011, 108(23):9384-9389. [33] MACDONALD L H, MOON H S, JAFFÉ P R. The role of biomass, electron shuttles, and ferrous iron in the kinetics of Geobacter sulfurreducens-mediated ferrihydrite reduction[J]. Water Research, 2011, 45(3):1049-1062. [34] RATASUK N, NANNY M A. Characterization and quantification of reversible redox sites in humic substances[J]. Environmental Science & Technology, 2007, 41(22):7844-7850. [35] BAUER I, KAPPLER A. Rates and extent of reduction of Fe(Ⅲ) compounds and O2 by humic substances[J]. Environmental Science & Technology, 2009, 43(13):4902-4908. [36] 袁英,何小松,席北斗,等. 腐殖质氧化还原和电子转移特性研究进展[J]. 环境化学,2014,33(12):2048-2057. YUAN Y, HE X S, XI B D, et al. Research progress on the redox and electron transfer capacity of humic substances[J]. Environmental Chemistry, 2014, 33(12):2048-2057(in Chinese).
[37] DUNNIVANT F M, SCHWARZENBACH R P, MACALADY D L. Reduction of substituted nitrobenzene in aqueous solutions containing natural organic matter[J]. Environmental Science & Technology, 1992, 26:2133-2141. [38] TRATNYEK P G and MACALADY D L. Abiotic reduction of nitro aromatic pesticides in anaerobic laboratory systems[J]. Journal of Agricultural and Food Chemistry, 1989, 37:248-254. [39] SCOTT D T, MCKNIGHT D M, BLUNT-HARRIS E L, et al. Quinone moieties act as electron acceptors in the reduction of humic substances by humics-reducing microorganisms[J]. Environmental Science & Technology, 1998, 32(19):2984-2989. [40] NEWMAN D K, KOLTER R. A role for excreted quinones in extracellular electron transfer[J]. Nature, 2000, 405(6782):94-97. [41] SPOSITO G. Electron shuttling by natural organic matter:twenty years after. In Aquatic Redox Chemistry[M]. New York:Oxford University Press, 2011, 1071:113-127. [42] HERNÁNDEZ-MONTOYA V, ALVAREZ L H, MONTES-MORÁN M A, et al. Reduction of quinone and non-quinone redox functional groups in different humic acid samples by Geobacter sulfurreducens[J]. Geoderma, 2012, 183-184:25-31. [43] FIMMEN R L, CORY R M, CHIN Y P, et al. Probing the oxidation-reduction properties of terrestrially and microbially derived dissolved organic matter[J]. Geochimica et Cosmochimica Acta, 2007, 71(12):3003-3015. [44] SPOSITO G, STRUYK Z. Redox properties of standard humic acids[J]. Geoderma, 2001, 102(3-4):329-346. [45] WOLF M, KAPPLER A, JIANG J, et al. Effects of humic substances and quinones at low concentrations on ferrihydrite reduction by Geobacter metallireducens[J]. Environmental Science & Technology, 2009, 43(15):5679-5685. [46] 姜杰,李黎,孙国新. 基于三维荧光光谱特征研究土壤腐殖质氧化还原特性[J]. 环境化学,2012,31(12):2002-2006. JIANG J, LI L, SUN G X. Investigation of redox activities of soil humic acids using 3D excitation emission matrix fluorescence spectroscopy[J]. Environmental Chemistry, 2012, 31(12):2002-2006(in Chinese).
[47] OSTERBERG R, SHIRSHOVA L. Oscillating, nonequilibrium redox properties of humic acids[J]. Geochimica et Cosmochimica Acta, 1997, 61(21):4599-4604. [48] STRAUB K L, BENZ M, SCHINK B. Iron metabolism in anoxic environments at near neutral pH[J]. FEMS Microbiology Ecology, 2001, 34(3):181-186. [49] LIU C, ZACHARA J M, FOSTER N S, et al. Kinetics of reductive dissolution of hematite by bioreduced anthraquinone-2,6-disulfonate[J]. Environmental Science & Technology, 2007, 41(22):7730-7735. [50] AESCHBACHER. Electrochemical redox characterization of humic substances[D]. Switzerland:ETH Zurich, 2011. [51] GAMAGE R, MCQUILLAN A J, PEAKE B M. Ultraviolet-visible and electron paramagnetic resonance spectroelectrochemical studies of the reduction products of some anthraquinone sulphonates in aqueous solutions[J]. Journal of the Chemical Society, Faraday Transactions, 1991, 87(22):3653-3660. [52] KLVPFEL L. Redox characteristics of quinones in natural organic matter(NOM)[D]. Switzerland:Term paper FS 2009, Institute of Biogeochemistry and Pollutant Dynamics ETH Zürich. [53] GU B H, CHEN J. Enhanced microbial reduction of Cr(Ⅵ) and U(Ⅵ) by different natural organic matter fractions[J]. Geochimica et Cosmochimica Acta, 2003, 67(19):3575-3582. [54] CERVANTES F J, GUTIÉRREZ C H, LÓPEZ K Y, et al. Contribution of quinone-reducing microorganisms on the anaerobic biodegradation of organic compounds under different redox conditions[J]. Biodegradation, 2008, 19(2):235-246. [55] SHARPLESS C M, AESCHBACHER M, PAGE S E, et al. Photooxidation-induced changes in optical, electrochemical and photochemical properties of humic substances[J]. Environmental Science & Technology, 2014, 48(5):2688-2696. [56] GRAY H B, WINKLER J R. Long-range electron transfer[J]. Proceedings of the National Academy of Sciences, USA, 2005, 102(10):3534-3539. [57] CERVANTES F J, DE BOK F A M, TUAN D D, et al. Reduction of humic substances by halorespiring, sulphatereducing and methanogenic microorganisms[J]. Environmental Microbiology, 2002, 4(1):51-57. [58] AMSTAETTER K, BORCH T, KAPPLER A. Influence of humic acid imposed changes of ferrihydrite aggregation on microbial Fe(Ⅲ) reduction[J]. Geochimica et Cosmochimica Acta, 2012, 85:326-341. [59] PIEPENBROCK A, DIPPON U, PORSCH K, et al. Dependence of microbial magnetite formation on humic substance and ferrihydrite concentrations[J]. Geochimica et Cosmochimica Acta, 2011, 75(22):6844-6858. [60] JIANG J, BAUER I, PAUL A, et al. Arsenic redox changes by microbially and chemically formed semiquinone radicals and hydroquinones in a humic substance model quinone[J]. Environmental Science & Technology, 2009, 43(10):3639-3645. [61] AESCHBACHER M, VERGARY D, SCHWARZENBACH R P, et al. Electrochemical analysis of proton and electron transfer equilibria of the reducible moieties in humic acids[J]. Environmental Science & Technology, 2011, 45(19):8385-8394. [62] LOVLEY D R, HOLMES D E, NEVIN K P. Dissimilatory Fe(Ⅲ) and Mn(Ⅳ) reduction. In Advances in Microbial Physiology[M]. New York:Academic Press, 2004, 49:219-286. [63] LU N, ZHOU S G, NI J R. Mechanism of energy generation of microbial fuel cells[J]. Progress in Chemistry, 2008, 20(7-8):1233-1240. [64] TURIEK C E, TISA L S, CACCAVO F. Melanin production and use as a soluble electron shuttle for Fe(Ⅲ) oxide reduction and as a terminal electron acceptor by Shewanella alga BrY[J]. Applied and Environment Microbiology, 2002, 68(5):2436-2444. [65] HERNANDEZ M E, KAPPLER A, NEWMAN D K. Phenazines and other redox-active antibiotics promote microbial mineral reduction[J]. Applied and Environment Microbiology, 2004, 70(2):921-928. [66] KEILUWEIT M, NICO P S, JOHNSON M G, et al. Dynamic molecular structure of plant biomass-derived black carbon(biochar)[J]. Environmental Science & Technology, 2010, 44(4):1247-1253. [67] HEYMANN K, LEHMANN J, SOLOMON D, et al. C1s K-edge near edge X-ray absorption fine structure(NEXAFS) spectroscopy for characterizing functional group chemistry of black carbon[J]. Organic Geochemistry, 2011, 42(9):1055-1064. [68] KLVPFEL L, KEILUWEIT M, KLEBER M, et al. Redox properties of plant biomass-derived black carbon(biochar)[J]. Environmental Science & Technology, 2014, 48(1):5601-5611. [69] TONG H, HU M, LI F B, et al. Biochar enhances the microbial and chemical transformation of pentachlorophenol in paddy soil[J]. Soil Biology & Biochemistry, 2014, 70:142-150. [70] XU W, PIGNATELLO J J, MITCH W A. Role of black carbon electrical conductivity in mediating hexahydro-1,3,5-trinitro-1,3,5-triazine(RDX) transformation on carbon surfaces by sulfides[J]. Environmental Science & Technology, 2013, 47(13):7129-7136. [71] KAPPLER A, WUESTNER M L, RUECKER A, et al. Biochar as an electron shuttle between bacteria and Fe(Ⅲ) minerals[J]. Environmental Science & Technology Lett, 2014, 1(8):339-344. [72] 窦森. 土壤有机质[M]. 北京:科学出版社,2010. DOU S. Soil organic matter[M]. Beijing:Science Press, 2010(in Chinese). [73] SCHMIDT M W I, TORN M S, ABIVEN S, et al. Persistence of soil organic matter as an ecosystem property[J]. Nature, 2011, 478(7367):49-56. [74] KELLEHER B P, SIMPSON A J. Humic substances in soils:Are they really chemically distinct[J]. Environmental Science & Technology, 2006, 40(15):4605-4611. [75] LEHMANN J, SOLOMON D, KINYANGI J, et al. Spatial complexity of soil organic matter forms at nanometer scales[J]. Nature Geoscience, 2008, 1(4):238-242. [76] KLEBER M, JOHNSON M G. Advances in understanding the molecular structure of soil organic matter:implications for interactions in the environment. In Advances in Agronomy[M]. San Diego:Elsevier Academic Press Inc, 2010, 106:77-142. [77] NIELSEN L P, RISGAARD-PETERSEN N, FOSSING H, et al. Electric currents couple spatially separated biogeochemical processes in marine sediment[J]. Nature, 2010, 463(7284):1071-1074. [78] FULDA B, VOEGELIN A, MAURER F, et al. Copper redox transformation and complexation by reduced and reoxidized soil humic acid. 1. X-ray absorption spectroscopy study[J]. Environmental Science & Technology, 2013, 47(19):10903-10911. [79] MAURER F, CHRISTL I, FULDA B, et al. Copper redox transformation and complexation by reduced and oxidized soil humic acid. 2. Potentiometric titrations and dialysis cell experiments[J]. Environmental Science & Technology, 2013, 47(19):10912-10921. [80] RAKSHIT S, UCHIMIYA M, SPOSITO G. Iron(Ⅲ) bioreduction in soil in the presence of added humic substances[J]. Soil Science Society of America Journal, 2009, 73(1):65-71. [81] TUO Y, LIU G, ZHOU J, et al. Microbial formation of palladium nanoparticles by Geobacter sulfurreducens for chromate reduction[J]. Bioresource Technology, 2013, 133:606-611. [82] 江韬,魏世强,李雪梅,等. 胡敏酸对汞还原能力的测定和表征[J]. 环境科学,2012,33(1):286-292. JIANG T, WEI S Q, LI X M, et al. Determination and characterization on the capacity of humic acid for the reduction of divalent mercury[J]. Environmental Science, 2012, 33(1):286-292(in Chinese).
[83] 徐丽娜,李忠佩,车玉萍. 淹水厌氧条件下腐殖酸对红壤中铁异化还原过程的影响[J]. 环境科学,2009,30(1):221-226. XU L N, LI Z P, CHE Y P. Influences of humic acids on the dissimilatory iron reduction of red soil in anaerobic condition[J]. Environmental Science, 2009, 30(1):221-226(in Chinese).
[84] MAURER F, CHRISTL I, HOFFMANN M, et al. Reduction and reoxidation of humic acid:Influence on speciation of cadmium and sliver[J]. Environmental Science & Technology, 2012, 46(16):8808-8816. [85] SKOGERBOE R K, WILSON S A. Reduction of ionic species by fulvic acid[J]. Analytical Chemistry, 1981, 53(2):228-232. [86] WITTBRODT P R, PALMER C D. Reduction of Cr(Ⅵ) in the presence of excess soil fulvic acid[J]. Environmental Science & Technology, 1995, 29(1):255-263. [87] WANG X, LIU G, ZHOU J, et al. Quinone-mediated reduction of selenite and tellurite by Escherichia coli[J]. Bioresource Technology, 2011, 102(3):3268-3271. [88] BRADLEY P M, CHAPELLE F H, LOVLEY D R. Humic acids as electron acceptors for anaerobic microbial oxidation of vinyl chloride and dichloroethene[J]. Applied and Environment Microbiology, 1998, 64(8):3102-3105. [89] CERVANTES F J, DIJKSMA W, DUONG-DAC T, et al. Anaerobic mineralization of toluene by enriched sediments with quinones and humus as terminal electron acceptors[J]. Applied and Environment Microbiology, 2001, 67(10):4471-4478. [90] CERVANTES F J, VAN DER ZEE F P, LETTINGA G, et al. Enhanced decolourisation of acid orange 7 in a continuous UASB reactor with quinones as redox mediators[J]. Water Science and Technology, 2001, 44(4):123-128. [91] ZHANG T, BAIN T S, NEVIN K P, et al. Anaerobic benzene oxidation by Geobacter species[J]. Applied and Environment Microbiology, 2012, 78(23):8304-8310. [92] MA C, WANG Y Q, ZHUANG L, et al. Anaerobic degradation of phenanthrene by a newly isolated humus-reducing bacterium, Pseudomonas aeruginosa strain PAH-1[J]. Journal of Soils and Sediments, 2011, 11(6):923-929. [93] CERVANTES F J, VAN DER VELDE S, LETTINGA G, et al. Quinones as terminal electron acceptors for anaerobic microbial oxidation of phenolic compounds[J]. Biodegradation, 2000, 11(5):313-321. [94] WEI N, FINNERAN K T. Microbial community analyses of three distinct, liquid cultures that degrade methyl tert-butyl ether using anaerobic metabolism[J]. Biodegradation, 2009, 20(5):695-707. [95] CERVANTES F J, GONZÁLEZ-ESTRELLA J, MÁRQUEZ A, et al. Immobilized humic substances on an anion exchange resin and their role on the redox biotransformation of contaminants[J]. Bioresource Technology, 2011, 102(2):2097-2100. [96] CERVANTES F J, VAN DER VELDE S, LETTINGA G, FIELD J A. Competition between methanogenesis and quinone respiration for ecologically important substrates in anaerobic consortia[J]. FEMS Microbiology Ecology, 2000, 34(2):161-171. [97] ARANDA-TAMAURA C, ESTRADA-ALVARADOl M I, et al. Effects of different quinoid redox mediators on the removal of sulphide and nitrate via denitrification[J]. Chemosphere, 2007, 69(11):1722-1727. [98] VAN DER ZEE F P, BOUWMAN R H M, STRIK D P B T B, et al. Application of redox mediators to accelerate the transformation of reactive azo dyes in anaerobic bioreactors[J]. Biotechnology and Bioengineering, 2001, 75(6):691-701. [99] WATANABE K, MANEFIELD M, LEE M, et al. Electron shuttles in biotechnology[J]. Current Opinion in Biotechnology, 2009, 20(6):633-641. [100] 许志诚,洪义国,罗微,等. 厌氧条件下希瓦氏菌腐殖质还原对偶氮还原的影响[J]. 微生物学报,2006,46(4):591-597. XU Z C, HONG Y G, LUO W, et al. The effects of the humic substances on azoreduction by Shewanella spp.[J]. Acta Microbiologica Sinica, 2006, 46(4):591-597(in Chinese).
[101] GUO J, ZHOU J, WANG D, et al. Biocatalyst effects of immobilized anthraquinone on the anaerobic reduction of azo dyes by the salt-tolerant bacteria[J]. Water Research, 2007. 41(2):426-432. [102] CERVANTES F J, MANCILLA A R, RIOS-DEL TORO E E, et al. Anaerobic benzene oxidation by enriched inocula with humic acids as terminal electron acceptors[J]. Journal of Hazardous Materials, 2011, 195:201-207. [103] YE X, ZHANG X, MORGENROTH E, et al. Exogenous anthrahydroquinone-2,6-disulfonate specifically increases xylose utilization during mixed sugar fermentation by Clostridium beijerinckii NCIMB 8052[J]. International Journal of Hydrogen Energy, 2013, 38(6):2719-2727. [104] YE X, MORGENROTH E, ZHANG X, et al. Anthrahydroquinone-2,6-disulfonate(AH2QDS) increases hydrogen molar yield and xylose utilization in growing cultures of Clostridium beijerinckii[J]. Applied Microbiology & Biotechnology, 2011, 92(4):855-864. [105] ZHANG X, YE X, GUO B, et al. Lignocellulosic hydrolysates and extracellular electron shuttles for H2 production using co-culture fermentation with Clostridium beijerinckii and Geobacter metallireducens[J]. Bioresource Technology 2013, 147:89-95. [106] 王慧勇,梁鹏,黄霞,等. 微生物燃料电池中产电微生物电子传递研究进展[J]. 环境保护科学,2009,35(1):17-20 ,35. WANG H Y, LIANG P, HUANG X, et al. Research progress in microbial fuel cells for electrons transfer of electricigens[J]. Environmental Protection Science, 2009, 35(1):17-20, 35(in Chinese).
[107] RINGEISEN B R, HENDERSON E, WU P K, et al. High power density from a miniature microbial fuel cell using Shewanella oneidensis DSP10[J]. Environmental Science & Technology, 2006, 40(8):2629-2634. [108] THYGESEN A, POULSEN F W, MIN B, et al. The effect of different substrates and humic acid on power generation in microbial fuel cell operation[J]. Bioresource Technology, 2009, 100(3):1186-1191. [109] WANG Y, ZHOU D, WANG Y, et al. Humic acid and metal ions accelerating the dechlorination of 4-chlorobiphenyl by nanoscale zero-valent iron[J]. Environmental Science, 2011, 23(8):1286-1292. [110] FORREZ I, CARBALLA M, FINK G, et al. Biogenic metals for the oxidative and reductive removal of pharmaceuticals, biocides and iodinated contrast media in a polishingmembrane bioreactor[J]. Water Research, 2011, 45(4):1763-1773. -
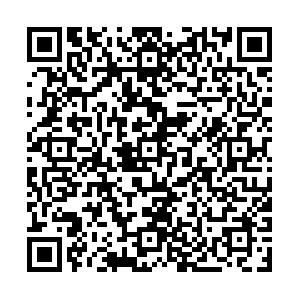
计量
- 文章访问数: 2745
- HTML全文浏览数: 2570
- PDF下载数: 1260
- 施引文献: 0