环境浓度氧化石墨烯和多环芳烃复合暴露诱发成年斑马鱼脑组织的分子响应研究
Molecular Response in Adult Zebrafish Brain Induced by Environment-related Concentration Graphene Oxide and Polycyclic Aromatic Hydrocarbons
-
摘要: 氧化石墨烯(graphene oxide,GO)是目前十分重要的人工纳米材料,且极有可能被排放到环境中。因而GO与环境中的共存污染物多环芳烃(polycyclic aromatic hydrocarbons,PAHs)对水生生物的复合暴露毒性及其相关机理值得进一步研究。使用成年斑马鱼为动物模型,选取水环境浓度的典型污染物16种优控PAHs与环境预测浓度的GO对成年斑马鱼进行21 d亚急性暴露,研究GO和PAHs复合暴露对成年斑马鱼脑组织的毒性效应及其分子机理。结果表明,GO暴露组和PAHs-GO复合暴露组在21 d的亚急性暴露中均不会诱发成年斑马鱼的死亡和畸形,但会降低斑马鱼脑组织细胞色素P4501B1酶(CYP1B1)和β-半乳糖苷酶(β-Gal)的含量;在分子水平上,0.1 mg·L-1 GO组(GO组)、5 μg·L-1 PAHs组(PAHs组)和0.1 mg·L-1 GO&5 μg·L-1 PAHs组(PAHs-GO组)诱发的差异基因数量和差异基因通路数量排序为PAHs组>GO-PAHs组>GO组。研究表明:(1)在环境预测浓度的GO与环境浓度PAHs对成年斑马鱼复合暴露时,对斑马鱼脑组织酶的影响GO占据主导地位;(2) PAHs-GO复合暴露组兼具GO和PAHs的分子毒性效应的特点,说明GO和PAHs在环境相关浓度下的复合暴露分子毒性值得注意;(3) GO组转录组氧化酶相关基因变化与酶含量结果相一致,暗示可以使用转录组来解释生物酶含量变化的分子机理。本文结果可为纳米材料与环境污染物的复合毒性效应和机理研究提供参考。Abstract: Currently, graphene oxide (GO), as an important engineering nanomaterial, has a high potential to enter the environment. Therefore, the combined ecological mechanism and health effects of GO and polycyclic aromatic hydrocarbons (PAHs, a coexisting pollutants) on aquatic organisms need further study. In this study, adult zebrafish was selected as animal model for sub-acute exposure toxic research of GO at predicted environment concentration and 16 priority PAHs (typical pollutants) at environment concentration after 21 d of exposure. Then, combined toxic effects and molecular mechanism of GO and PAHs on adult zebrafish brain were explored. Results showed that both GO and PAHs-GO groups didn't induce death and malformation in adult zebrafish during 21 d of subacute exposure, but decreased cytochrome P4501B1 (CYP1B1) and β-galactosidase (β-Gal) content in zebrafish brain. Based on differential expressed genes (DEGs) and altered function pathways induced by treatment groups, the alteration order at the molecular level induced by 0.1 mg·L-1 GO (GO group), 5 μg·L-1 PAHs (PAHs group), and 0.1 mg·L-1 GO & 5 μg·L-1 PAHs (PAHs-GO group) was PAHs group > PAHs-GO group > GO group. In summary, this study reveals that (1) GO play a dominant role in alteration of CYP1B1 and β-Gal content in adult zebrafish brain for 21 d exposure to GO and co-exposure to GO with PAHs; (2) PAHs-GO co-exposure group share a common characteristic in the toxic effects of both GO and PAHs group, indicating the potential toxicity induced by PAHs-GO at environmental related concentration co-exposure need to notice; (3) For GO group, the alteration of oxidase content were consistent with the transcriptomic result, indicating that transcriptomics may help explain the molecular mechanism of oxidase alteration induced by GO. The results of this study can provide a reference for the combined toxicity effect and mechanism of nanomaterials and environmental coexisting pollutants.
-
Key words:
- graphene oxide /
- polycyclic aromatic hydrocarbons /
- zebrafish /
- sub-acute toxicity /
- transcriptome
-
-
Goodwin D G Jr, Adeleye A S, Sung L, et al. Detection and quantification of graphene-family nanomaterials in the environment[J]. Environmental Science & Technology, 2018, 52(8):4491-4513 Hu X G, Zhou Q X. Health and ecosystem risks of graphene[J]. Chemical Reviews, 2013, 113(5):3815-3835 Liu Y, Nie Y G, Wang J J, et al. Mechanisms involved in the impact of engineered nanomaterials on the joint toxicity with environmental pollutants[J]. Ecotoxicology and Environmental Safety, 2018, 162:92-102 Wang Y, Comer J, Chen Z F, et al. Exploring adsorption of neutral aromatic pollutants onto graphene nanomaterials via molecular dynamics simulations and theoretical linear solvation energy relationships[J]. Environmental Science:Nano, 2018, 5(9):2117-2128 Sun Y B, Yang S B, Zhao G X, et al. Adsorption of polycyclic aromatic hydrocarbons on graphene oxides and reduced graphene oxides[J]. Chemistry, An Asian Journal, 2013, 8(11):2755-2761 Zhou Q X, Hu X G. Systemic stress and recovery patterns of rice roots in response to graphene oxide nanosheets[J]. Environmental Science & Technology, 2017, 51(4):2022-2030 Bragin G E, Parkerton T F, Redman A D, et al. Chronic toxicity of selected polycyclic aromatic hydrocarbons to algae and crustaceans using passive dosing[J]. Environmental Toxicology and Chemistry, 2016, 35(12):2948-2957 Bezza F A, Chirwa E M N. The role of lipopeptide biosurfactant on microbial remediation of aged polycyclic aromatic hydrocarbons (PAHs)-contaminated soil[J]. Chemical Engineering Journal, 2017, 309:563-576 范博, 王晓南, 黄云, 等. 我国七大流域水体多环芳烃的分布特征及风险评价[J]. 环境科学, 2019, 40(5):2101-2114 Fan B, Wang X N, Huang Y, et al. Distribution and risk assessment of polycyclic aromatic hydrocarbons in water bodies in seven basins of China[J]. Environmental Science, 2019, 40(5):2101-2114(in Chinese)
Tang Y M, Junaid M, Niu A P, et al. Diverse toxicological risks of PAHs in surface water with an impounding level of 175m in the Three Gorges Reservoir Area, China[J]. The Science of the Total Environment, 2017, 580:1085-1096 Yan J X, Liu J L, Shi X, et al. Polycyclic aromatic hydrocarbons (PAHs) in water from three estuaries of China:Distribution, seasonal variations and ecological risk assessment[J]. Marine Pollution Bulletin, 2016, 109(1):471-479 Zhou Q X, Cheng Y, Zhang Q R, et al. Quantitative analyses of relationships between ecotoxicological effects and combined pollution[J]. Science in China Series C, Life Sciences, 2004, 47(4):332-339 Linard E N, Apul O G, Karanfil T, et al. Bioavailability of carbon nanomaterial-adsorbed polycyclic aromatic hydrocarbons to Pimphales promelas:Influence of adsorbate molecular size and configuration[J]. Environmental Science & Technology, 2017, 51(16):9288-9296 Zindler F, Glomstad B, Altin D, et al. Phenanthrene bioavailability and toxicity to Daphnia magna in the presence of carbon nanotubes with different physicochemical properties[J]. Environmental Science & Technology, 2016, 50(22):12446-12454 Wang Z, Zhang F, Wang S, et al. Assessment and prediction of joint algal toxicity of binary mixtures of graphene and ionic liquids[J]. Chemosphere, 2017, 185:681-689 Garcia G R, Noyes P D, Tanguay R L. Advancements in zebrafish applications for 21st Century toxicology[J]. Pharmacology & Therapeutics, 2016, 161:11-21 Audira G, Lee J S, Siregar P, et al. Comparison of the chronic toxicities of graphene and graphene oxide toward adult zebrafish by using biochemical and phenomic approaches[J]. Environmental Pollution, 2021, 278:116907 Alexandre-Moreno S, Bonet-Fernández J M, Atienzar-Aroca R, et al. Null cyp1b1 activity in zebrafish leads to variable craniofacial defects associated with altered expression of extracellular matrix and lipid metabolism genes[J]. International Journal of Molecular Sciences, 2021, 22(12):6430 Mu L, Gao Y, Hu X G. Characterization of biological secretions binding to graphene oxide in water and the specific toxicological mechanisms[J]. Environmental Science & Technology, 2016, 50(16):8530-8537 Zhang X L, Zhou Q X, Zou W, et al. Molecular mechanisms of developmental toxicity induced by graphene oxide at predicted environmental concentrations[J]. Environmental Science & Technology, 2017, 51(14):7861-7871 孙晶, 欧阳少虎, 胡献刚, 等. 3种碳纳米材料对斑马鱼生长发育、氧化应激及代谢的影响[J]. 生态毒理学报, 2020, 15(6):101-114 Sun J, Ouyang S H, Hu X G, et al. Effects of three carbonaceous nanomaterials on the developmental toxicity, oxidative stress, and metabolic profile in zebrafish[J]. Asian Journal of Ecotoxicology, 2020, 15(6):101-114(in Chinese)
Li X K, Mu L, Hu X G. Integrating proteomics, metabolomics and typical analysis to investigate the uptake and oxidative stress of graphene oxide and polycyclic aromatic hydrocarbons[J]. Environmental Science:Nano, 2018, 5(1):115-129 Nouara A, Wu Q L, Li Y X, et al. Carboxylic acid functionalization prevents the translocation of multi-walled carbon nanotubes at predicted environmentally relevant concentrations into targeted organs of nematode Caenorhabditis elegans[J]. Nanoscale, 2013, 5(13):6088-6096 Zou W, Zhou Q X, Zhang X L, et al. Characterization of the effects of trace concentrations of graphene oxide on zebrafish larvae through proteomic and standard methods[J]. Ecotoxicology and Environmental Safety, 2018, 159:221-231 Sun J, Zhou Q X, Hu X G. Integrating multi-omics and regular analyses identifies the molecular responses of zebrafish brains to graphene oxide:Perspectives in environmental criteria[J]. Ecotoxicology and Environmental Safety, 2019, 180:269-279 Cooper R J, Menking-Colby M N, Humphrey K A, et al. Involvement of β-catenin in cytoskeleton disruption following adult neural stem cell exposure to low-level silver nanoparticles[J]. Neurotoxicology, 2019, 71:102-112 Fayeulle A, Veignie E, Slomianny C, et al. Energy-dependent uptake of benzo[a] pyrene and its cytoskeleton-dependent intracellular transport by the telluric fungus Fusarium solani[J]. Environmental Science and Pollution Research International, 2014, 21(5):3515-3523 Sforzini S, Oliveri C, Orrù A, et al. Application of a new targeted low density microarray and conventional biomarkers to evaluate the health status of marine mussels:A field study in Sardinian coast, Italy[J]. The Science of the Total Environment, 2018, 628-629:319-328 Ferreira J L R, Lonné M N, França T A, et al. Co-exposure of the organic nanomaterial fullerene C60 with benzo[a] pyrene in Danio rerio (zebrafish) hepatocytes:Evidence of toxicological interactions[J]. Aquatic Toxicology, 2014, 147:76-83 Baun A, Sørensen S N, Rasmussen R F, et al. Toxicity and bioaccumulation of xenobiotic organic compounds in the presence of aqueous suspensions of aggregates of nano-C(60)[J]. Aquatic Toxicology, 2008, 86(3):379-387 Geiger B, Nguyen H M, Wenig S, et al. From by-product to valuable components:Efficient enzymatic conversion of lactose in whey using β-galactosidase from Streptococcus thermophilus[J]. Biochemical Engineering Journal, 2016, 116:45-53 Geng Y Q, Guan J T, Xu X H, et al. Senescence-associated beta-galactosidase activity expression in aging hippocampal neurons[J]. Biochemical and Biophysical Research Communications, 2010, 396(4):866-869 Kim E J, Podder A, Maiti M, et al. Selective monitoring of vascular cell senescence via β-galactosidase detection with a fluorescent chemosensor[J]. Sensors and Actuators B:Chemical, 2018, 274:194-200 Geier M C, Chlebowski A C, Truong L, et al. Comparative developmental toxicity of a comprehensive suite of polycyclic aromatic hydrocarbons[J]. Archives of Toxicology, 2018, 92(2):571-586 Shimada T, Fujii-Kuriyama Y. Metabolic activation of polycyclic aromatic hydrocarbons to carcinogens by cytochromes P4501A1 and 1B1[J]. Cancer Science, 2004, 95(1):1-6 van Leeuwen L M, Evans R J, Jim K K, et al. A transgenic zebrafish model for the in vivo study of the blood and choroid plexus brain barriers using claudin 5[J]. Biology Open, 2018, 7(2):bio030494 Zhang J J, Liss M, Wolburg H, et al. Involvement of claudins in zebrafish brain ventricle morphogenesis[J]. Annals of the New York Academy of Sciences, 2012, 1257:193-198 Henkler F, Stolpmann K, Luch A. Exposure to polycyclic aromatic hydrocarbons:Bulky DNA adducts and cellular responses[J]. Experientia Supplementum, 2012, 101:107-131 Chen M J, Yin J F, Liang Y, et al. Oxidative stress and immunotoxicity induced by graphene oxide in zebrafish[J]. Aquatic Toxicology, 2016, 174:54-60 -
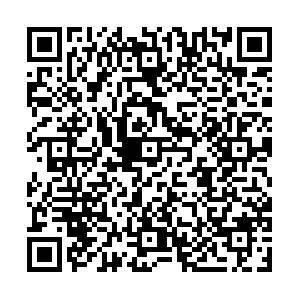
计量
- 文章访问数: 2466
- HTML全文浏览数: 2466
- PDF下载数: 87
- 施引文献: 0